The story of light dispersion through a prism begins with the foundational work of Sir Isaac Newton in the 17th century. Newton was fascinated by the nature of light and its properties. His curiosity led to a series of experiments that would forever change our understanding of optics.
In 1666, Newton conducted an experiment that was simple yet revolutionary. He allowed a beam of sunlight to pass through a triangular glass prism in a darkened room. To his surprise, instead of white light emerging from the other side, he observed a spectrum of colors.
Newton deduced that white light was not pure but a mixture of different colors. Each color had a different wavelength and bent by a different amount when passing through the prism. This bending, or refraction, caused the white light to spread out into a spectrum of colors, a phenomenon he named the dispersion of light.
Newton was meticulous and cautious about sharing his findings. It wasn’t until 1704, after much persuasion, that he published his work in a book titled “Opticks”. In it, he detailed his experiments and theories on light, including the concept of dispersion. Newton’s work laid the groundwork for modern optics. His prism experiment was a pivotal moment in science, leading to the development of new optical instruments and enhancing our understanding of the nature of light.
Today, Newton’s insights into the dispersion of light continue to influence various fields, from spectroscopy to astronomy. His principles are taught worldwide, forming a fundamental part of physics education.
What is the Dispersion of Light?
Dispersion of light is a fascinating and colorful phenomenon that occurs when white light is separated into its constituent colors. Dispersion of light is the separation of white light into its constituent colors when it passes through a medium like a prism. This occurs because different colors of light bend by different amounts due to their varying wavelengths.
Imagine white light as a team of runners, each wearing a different color shirt, racing through a medium like glass or water. As they run, they encounter a hurdle—the prism—which slows them down. But here’s the catch: each runner is slowed down by a different amount because of their unique shirt color, which represents the light’s wavelength.
In more scientific terms, dispersion happens because light is made up of waves, and these waves have different lengths. When white light enters a prism, each color of light is refracted, or bent, to a different degree. Violet light, with the shortest wavelength, is bent the most, and red light, with the longest wavelength, is bent the least. This separation of colors is what we call dispersion.
It’s like a musical band where each instrument plays a different note, and when they enter the prism, each note is directed to a different part of the room. The result is not just a single melody but a spectrum of musical notes spread across the space, similar to how light spreads across a spectrum of colors.
So, when we talk about the dispersion of light, we’re referring to this process of separating white light into a rainbow of colors. It’s a fundamental concept that explains why we see the colors we do and is essential for understanding the behavior of light as it travels through different mediums.
Dispersion of White Light by Glass Prism
The dispersion of white light by a glass prism occurs because different colors of light are refracted by different amounts due to their wavelengths. This results in the spread of white light into a continuous spectrum of colors, which we can observe as it exits the prism. When white light enters a glass prism, it refracts and splits into a spectrum of colors—red, orange, yellow, green, blue, indigo, and violet. Red light bends the least, while violet bends the most.
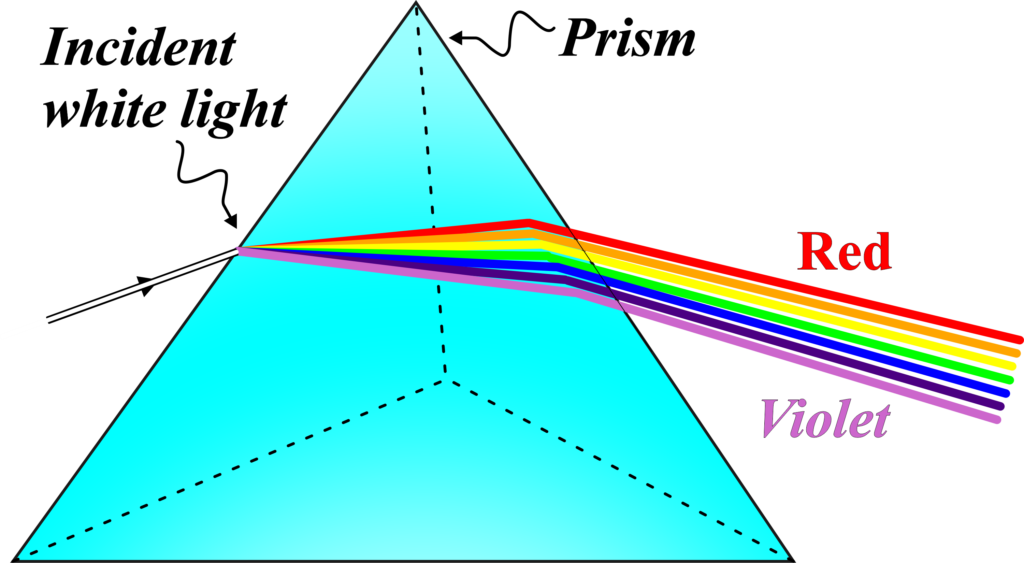
Imagine you have a beam of white light, like sunlight. This light contains all the colors of the rainbow, but you can’t see them because they’re all mixed. Now, let’s say you pass this white light through a triangular glass prism. What happens inside the prism is quite remarkable and is the essence of dispersion.
As the white light enters the prism, it encounters a change in medium from air to glass. This causes the light to slow down and bend—a process known as refraction. However, not all colors of light bend the same amount. Violet light, with its shorter wavelength, bends the most, and red light, with its longer wavelength, bends the least. This difference in bending causes the white light to spread out into a spectrum of colors.
Inside the prism, each color of light travels at a different speed because the glass prism has a different refractive index for each wavelength. This is why the colors separate: each one is refracted at a slightly different angle. When the colors emerge out of the prism, they have fanned out into a beautiful spectrum, displaying all the colors from violet to red.
This separation of colors is what we call the dispersion of white light. It’s a simple yet profound demonstration that white light is made up of a spectrum of colors, and it’s the glass prism that acts as the tool to reveal this hidden secret.
Factors Influencing Dispersion of Light:
Dispersion is the process where white light separates into its component colors. This happens when light passes through a medium like a prism. But what determines how much each color spreads out? That’s where the factors influencing dispersion come into play.
Refractive Index: The refractive index of a medium is a measure of how much it can bend light. Materials with a higher refractive index will bend light more, causing greater dispersion. Think of it like running on a track; if the track suddenly becomes softer, you’ll slow down more and your path will bend.
The wavelength of Light: The wavelength of light is another crucial factor. Colors with shorter wavelengths (like blue and violet) are dispersed more than colors with longer wavelengths (like red). It’s similar to how a small car can make sharper turns compared to a long bus.
The angle of Incidence: The angle of incidence is the angle at which light hits the prism. This angle affects how much the light is bent inside the prism. If the light enters at a steeper angle, the dispersion will be more pronounced, just as a ball hitting a wall at a sharp angle bounces off further away.
Material Composition: The material composition of the prism also plays a role. Different materials have different capacities to disperse light. For example, a diamond prism will disperse light more than a glass prism because of its unique internal structure.
Prism Shape: Lastly, the shape of the prism itself can influence dispersion. A prism with a more acute angle will spread the colors out more than a prism with a wider angle. This is because the light spends more time inside the prism, which increases the effect of dispersion.
By understanding these factors, students can get a clearer picture of why dispersion occurs and how it behaves under different conditions.
Refraction of Light through Prism
Refraction is the bending of light as it passes from one transparent medium into another. This happens because light travels at different speeds in different mediums.
When a beam of white light hits a glass prism, it enters from the air (a rarer medium) into a glass (a denser medium). As it enters the glass, the light slows down and bends towards the normal line—a line perpendicular to the surface at the point of contact.
Once inside the prism, the light continues to travel, bending further as it passes through the glass. The amount of bending depends on the angle of incidence (the angle at which the light hits the prism) and the material’s refractive index (a number that describes how much the material can bend light).
As the light exits the prism, it moves from the glass back into the air. It speeds up again and bends away from the normal line. This change in speed and direction is what causes the light to refract. The path that the light takes through the prism is not straight. Instead, it’s a zigzag path due to the bending at both the entry and exit points. The overall effect is that the light has changed direction from its original path—this change is the angle of deviation.
Light bends because it follows the law of refraction, also known as Snell’s Law. This law states that the ratio of the sine of the angle of incidence to the sine of the angle of refraction is constant and is equal to the refractive index. Refraction of light through a prism is a process where light bends as it passes from one medium to another, changing speed due to the different optical densities. This bending is responsible for the path of light being altered as it travels through the prism.
Visible Light Spectrum
The visible light spectrum is the range of electromagnetic waves that we can see. Each color has a different wavelength, and together they make up the light that brightens our world and allows us to enjoy the beauty of a rainbow or a sunset. The visible light spectrum is the range of light wavelengths that the human eye can see, approximately from 380 nm (violet) to 750 nm (red).
The visible light spectrum is the portion of the electromagnetic spectrum that is visible to the human eye. It consists of a range of wavelengths that we perceive as different colors. The spectrum includes the colors red, orange, yellow, green, blue, indigo, and violet.
Each color in the visible spectrum has a specific wavelength range:
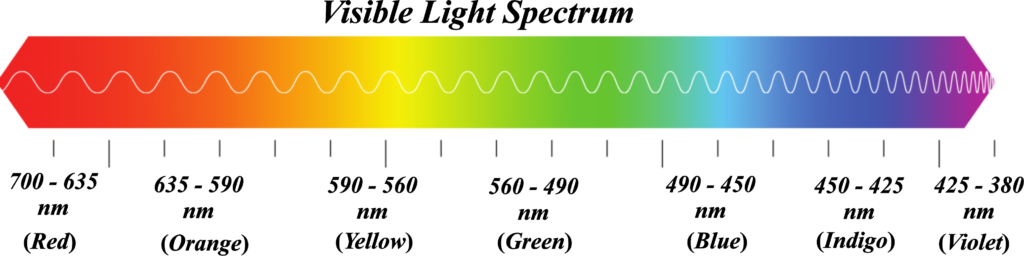
Our eyes have receptors called cones that are sensitive to these wavelengths. When light enters our eyes, the cones translate these wavelengths into electrical signals that our brain interprets as colors. The visible spectrum is continuous, meaning there are no gaps between the colors. They blend seamlessly into one another, creating the full array of colors we can see. Just outside the visible spectrum are ultraviolet light, which has shorter wavelengths, and infrared light, which has longer wavelengths. These are not visible to the naked eye but can be detected with special instruments.
Angle of Deviation
The angle of deviation is the angle between the direction of the incoming light and the direction of the light as it exits the prism. Each color has a different angle of deviation due to its unique wavelength. The angle of deviation is a measure used in optics to describe how much a beam of light is bent, or deviated, as it passes through a prism.
Imagine a football player running straight towards a goal. If the player encounters an obstacle that makes them change direction, the angle between their original path and their new path is similar to the angle of deviation in light. The greater the obstacle’s influence, the larger the deviation.
In technical terms, the angle of deviation (usually denoted by the Greek letter \(\displaystyle\delta \)) is the angle made between the incident ray of light entering the first face of the prism and the refracted ray that emerges from the second face of the prism. It’s like measuring the change in direction from where the light first hits the prism to where it comes out on the other side.
The angle of deviation is important because it tells us how much each color of light is bent by the prism. Since different colors of light have different wavelengths, they are deviated by different amounts. Violet light, for example, is bent more than red light.
The reason for this difference in bending is due to the light’s wavelength. Shorter wavelengths (like violet) are bent more, and longer wavelengths (like red) are bent less. This is why we see a spectrum of colors when white light passes through a prism – each color is deviated at a slightly different angle.
The exact angle of deviation depends on the prism’s material and the wavelength of light. It can be calculated using Snell’s Law and the prism’s geometry. For a simple glass prism, the formula to find the angle of deviation for a particular wavelength involves the refractive index of the material and the angle at which the light enters the prism.
The Angle of Deviation for White Light through a Prism:
The angle of deviation is the measure of how much a ray of light has been bent from its original path after passing through a prism. For white light, which is a mix of all visible colors, each color deviates by a different amount because each color has a different wavelength.
When white light enters a prism, it’s like a group of athletes, each running at a different speed, entering a field with a barrier. The athletes represent different colors of light, and the barrier represents the prism. As they cross the barrier, each one changes direction slightly. The amount they change direction, or their angle of deviation, depends on their speed, just like it depends on the wavelength of light.
Violet light, with the shortest wavelength, is bent the most, while red light, with the longest wavelength, is bent the least. This spread of colors is what creates a spectrum. The angle of deviation is largest for violet and smallest for red, with all the other colors falling in between.
The shape of the prism also affects the angle of deviation. A triangular prism with non-parallel sides will cause an overall angle of deviation for the various colors of white light, spreading them out to form a spectrum. The exact angle of deviation for each color can be calculated using Snell’s Law and the geometry of the prism.
Interestingly, there is a particular angle at which the light enters the prism where the deviation is the smallest for all colors. This is known as the angle of minimum deviation, and it occurs when the light travels symmetrically through the prism, with the path inside the prism being parallel to the base. This angle is unique for each color due to their different wavelengths.
The angle of deviation for white light through a prism is a beautiful demonstration of how light’s inherent properties, combined with the geometry of the prism, can lead to the colorful display we know as the spectrum.
Newton’s Prism Experiment
Sir Isaac Newton’s prism experiment was a pivotal moment in the scientific study of light and color. In 1666, Newton conducted a series of experiments to delve into the nature of light. Newton’s experiment showed that a prism could decompose white light into a spectrum of colors and that these colors could be recombined to form white light again.
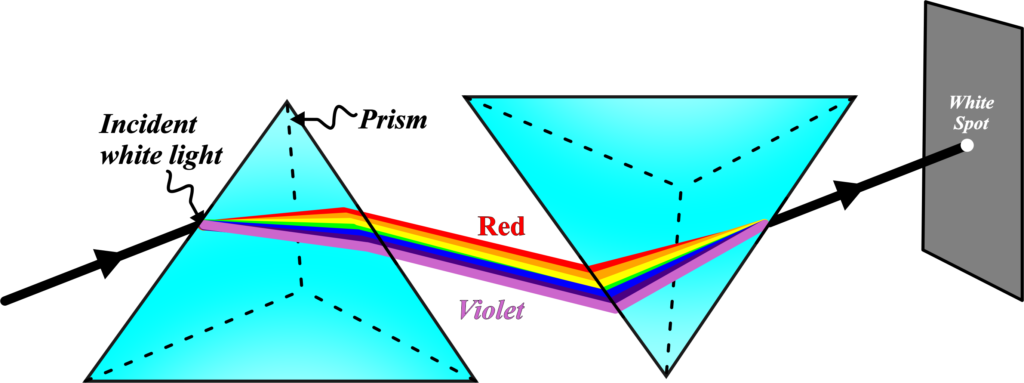
Newton darkened his room and made a small hole in his window shutter, allowing just a single beam of sunlight to enter. He then placed a triangular glass prism in the path of the sunlight. As the sunlight passed through the prism, it spread out into a band of colors on the opposite wall. This band, known as a spectrum, displayed all the colors of the rainbow: red, orange, yellow, green, blue, indigo, and violet.
Newton observed that the prism did not create the colors; rather, it separated the colors that were already present in the sunlight. He realized that white light is a mixture of all the colors of the visible spectrum. To confirm his hypothesis, Newton performed another experiment. He placed a second prism upside down in front of the spectrum created by the first prism. Instead of creating a new set of colors, the second prism recombined the spectrum back into white light.
Newton concluded that white light is composed of different colors, and these colors can be separated and recombined by refraction through prisms. His experiment fundamentally changed our understanding of light and laid the foundation for the field of optics. Newton’s experiment with prisms showed that light is a complex entity made up of various colors, which can be bent and separated by refraction. This experiment is a classic example of how simple observations can lead to profound scientific discoveries.
Also Read: Total Internal Reflection
Some Natural Phenomena Due to Sunlight
Rainbows
A rainbow is a perfect example of light dispersion that we can observe in the sky. It occurs when sunlight interacts with raindrops after a storm. When sunlight enters a raindrop, it slows down and bends—a process known as refraction. Because sunlight is made up of different colors, each color bends at a slightly different angle due to its unique wavelength. Violet light bends the most, and red light bends the least.
A rainbow is a spectacular example of sunlight being dispersed by water droplets in the atmosphere. This phenomenon occurs due to the combined effects of dispersion, refraction, and reflection of sunlight by the spherical water droplets of rain.
Conditions for Observing a Rainbow: The Sun must be shining in one part of the sky, for example, near the western horizon. It should be raining in the opposite part of the sky, such as the eastern horizon. An observer can only see a rainbow with their back facing the Sun.
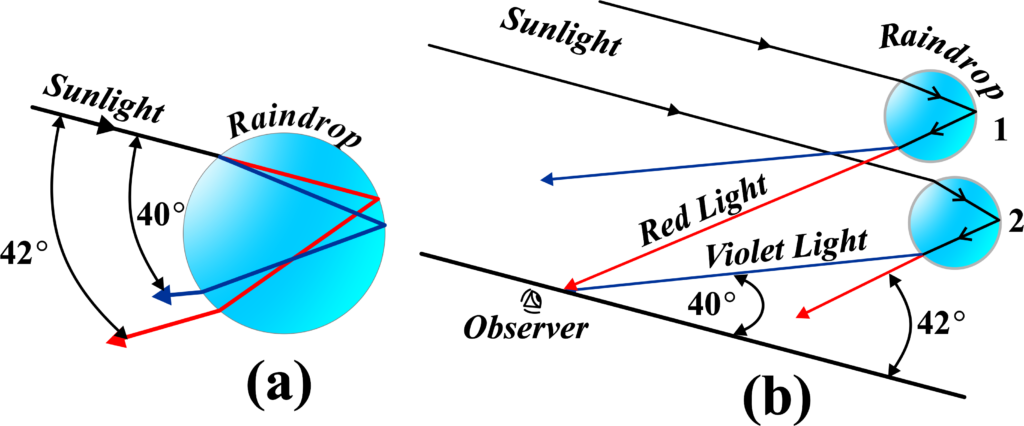
Figure (a) helps us understand the steps involved in the formation of a rainbow: When sunlight enters a raindrop, it is refracted (bent). This refraction causes the different wavelengths (colors) of white light to spread out. Longer wavelengths like red are bent the least, while shorter wavelengths like violet are bent the most.
These separated colors then strike the inner surface of the water droplet. If the angle of incidence is greater than the critical angle (48° for water), the light undergoes internal reflection.
The reflected light exits the droplet, undergoing another refraction. This further separates the colors. For example, violet light exits the raindrop at an angle of 40° relative to the incoming sunlight, and red light exits at an angle of 42°. Other colors emerge at angles between these two values.
Figure (b) shows how a primary rainbow forms: Red light from one droplet (drop 1) and violet light from another droplet (drop 2) reach the observer’s eye. Violet light from drop 1 and red light from drop 2 are directed above or below the observer.
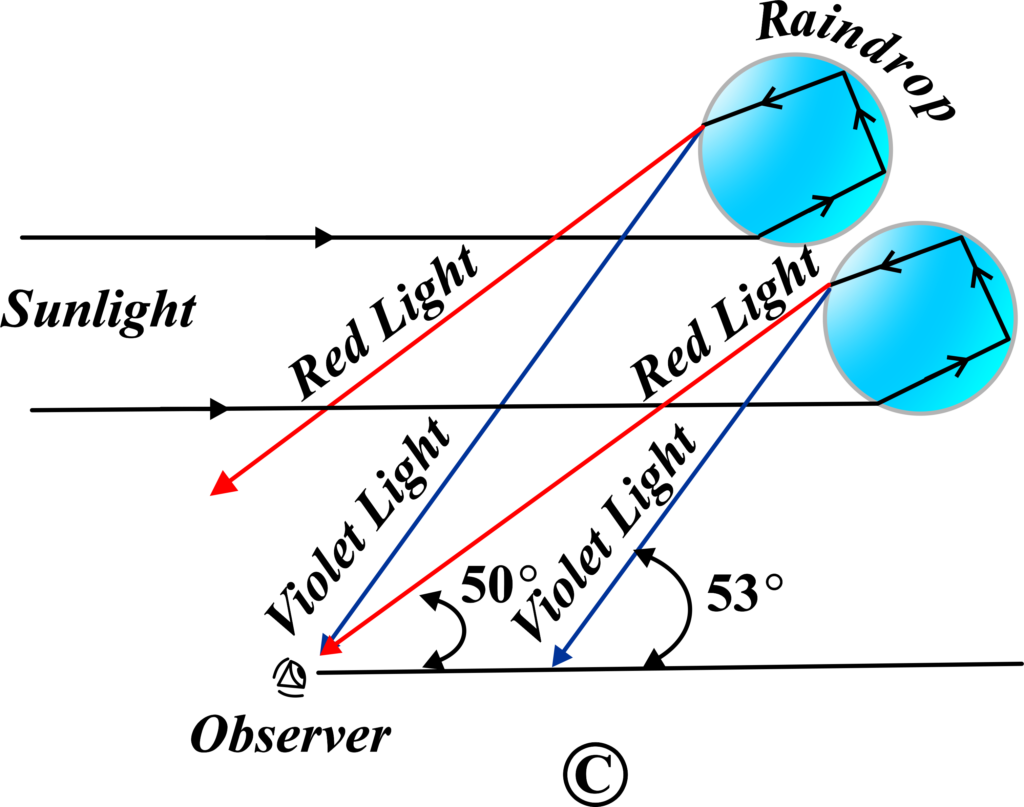
The observer sees a rainbow with red on the top and violet on the bottom because of the angles at which these colors emerge from the droplets. The primary rainbow results from a three-step process: refraction, reflection, and refraction.
Figure (c) explains the formation of a secondary rainbow: A secondary rainbow occurs when light undergoes two internal reflections inside a raindrop instead of one. The secondary rainbow is fainter because some light intensity is lost during the second reflection. The order of colors is reversed compared to the primary rainbow, with red on the inner edge and violet on the outer edge.
The arc shape of a rainbow is due to the angle at which the sunlight is refracted and then reflected inside the raindrops. The light that reaches your eyes from the higher raindrops appears red, while the light from the lower raindrops appears violet, with all the other colors in between.
The colors of a rainbow always appear in the same order: red, orange, yellow, green, blue, indigo, and violet. This sequence is determined by the wavelengths of the colors, with red having the longest wavelength and violet the shortest.
Scattering Of Light
Scattering of light occurs when light rays encounter small particles in the atmosphere, such as air molecules, dust, or water droplets. These particles cause the light to change direction and spread out. During sunset and sunrise, the sun is near the horizon, and sunlight travels through a longer path in the atmosphere. This longer journey means the light encounters more particles, leading to more scattering.
The phenomenon responsible for the scattering of light in the atmosphere is known as Rayleigh scattering. It’s more effective at shorter wavelengths, such as blue and violet light, which is why the sky appears blue during the day. However, at sunrise and sunset, the situation is a bit different.
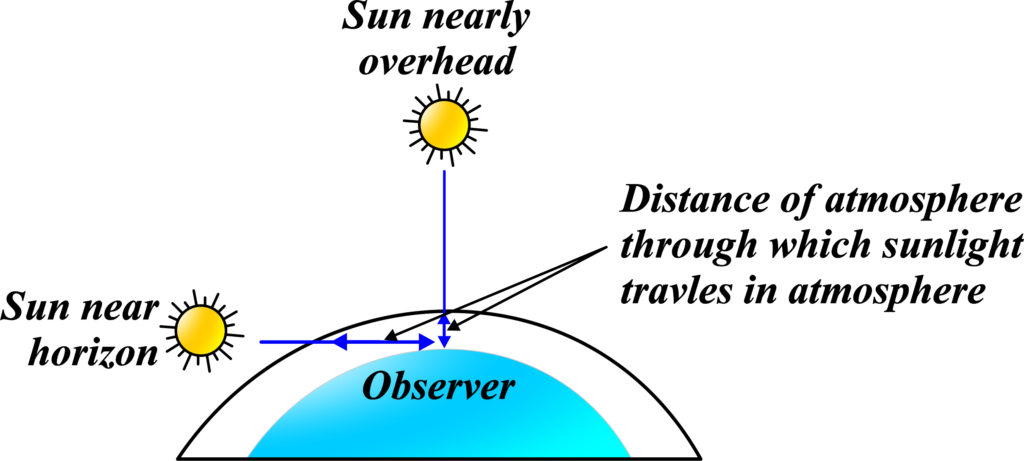
As the sun’s position is low, the sunlight’s path through the atmosphere is the longest. The blue and violet light is scattered out of the direct line of sight, and the longer wavelengths like red, orange, and yellow are less affected by scattering. This is why we often see a reddish sky at these times—the light that reaches us directly has more of the longer wavelengths.
Rayleigh’s law quantitatively describes the scattering of light, stating that the intensity of scattered light is inversely proportional to the fourth power of the wavelength. This means shorter wavelengths scatter much more than longer ones.
When light encounters particles in the atmosphere, it can be scattered. The nature of this scattering depends on the size of the particles relative to the wavelength of the light. The key factor here is the size of the particle (denoted as (a) compared to the wavelength of light (denoted as (λ). When the particles are much smaller than the wavelength of light (a << λ), we observe Rayleigh scattering.
Rayleigh scattering is more effective at shorter wavelengths. This is why the sky appears blue during the day; shorter (blue) wavelengths are scattered more than longer (red) wavelengths. The intensity of the scattered light (I) is inversely proportional to the fourth power of the wavelength (λ), which can be expressed as:
\(\displaystyle I \propto \frac{1}{\lambda^4} \)
For larger particles, such as dust and water droplets, the scattering is different. These particles are closer in size to the wavelength of visible light or even larger. In such cases, the scattering is less wavelength-dependent and can scatter light of all colors more or less equally. This is why clouds, which consist of water droplets, appear white; they scatter all wavelengths of light similarly.
Example: At sunset, sunlight travels through more of the Earth’s atmosphere, increasing the distance light travels through air and the number of particles it encounters. The increased scattering of shorter wavelengths of light (blue and violet) out of the line of sight leaves the longer wavelengths (red, orange, yellow) to reach the observer’s eyes, leading to the beautiful reds and oranges of a sunset.
The scattering of light in the atmosphere can vary depending on the size of the atmospheric particles relative to the wavelength of light. Rayleigh scattering dominates when the particles are much smaller than the wavelength, leading to the blue sky we see during the day, while larger particles scatter light more uniformly, contributing to the white appearance of clouds and the colors of sunsets.
Examples of Dispersion of Light
Rainbows: A rainbow is one of the most beautiful natural examples of light dispersion. After it rains, sunlight shines into droplets of water left in the air. Each droplet acts like a tiny prism, dispersing the sunlight into a spectrum of colors that arc across the sky.
Prisms: When white light passes through a glass prism, it is dispersed into a spectrum of colors. This happens because the different wavelengths of light are refracted by different amounts as they pass through the prism, separating the light into its constituent colors.
Soap Bubbles: The colorful patterns on soap bubbles are caused by dispersion. Light reflects off the different layers of soap film, and the varying thickness of the film causes different colors to be seen due to the dispersion of light.
CDs and DVDs: The surface of a CD or DVD can act like a prism. When light hits the surface, it’s dispersed into a rainbow of colors. This is due to the microscopic grooves on the disc diffracting the light and creating a spectrum.
Oil on Water: A thin layer of oil on water can create a rainbow effect. The light is dispersed by the varying thickness of the oil, which acts like a prism, separating the light into different colors.
These examples show how the dispersion of light is not just a concept in textbooks but a phenomenon that surrounds us, adding color to the natural world. It’s a principle that explains why we see such a variety of colors in different situations and helps students understand the practical implications of the theories they learn in class.
Practical Applications of Dispersion of Light
The dispersion of light, the process in which white light separates into its component colors, has several practical applications that are fascinating and integral to our daily lives. Here are some key applications explained simply:
- Spectroscopy: Spectroscopy is a technique that uses the dispersion of light to analyze the composition of materials. Scientists can identify the substance’s chemical makeup by passing light through a substance and examining the spectrum of colors produced.
- Optical Instruments: Prisms are used in various optical instruments like spectrometers and telescopes to disperse light into its constituent colors. This dispersion is crucial for understanding the properties of light from different celestial bodies.
- Vision Correction: Prism spectacles utilize dispersion to correct certain vision problems. These glasses have prisms that adjust the light entering the eyes, helping to correct alignment issues and improve visual clarity.
- Laser Tuning: In laser technology, dispersion is used to tune lasers to emit specific colors or wavelengths. This is essential in applications ranging from medical treatments to data transmission.
- Rainbow Formation: The natural phenomenon of rainbows is an example of dispersion. Sunlight disperses through water droplets in the atmosphere, creating the colorful arc we see in the sky after rain.
- Art and Decoration: The colorful patterns seen on CDs, soap bubbles, or oil spills on water are due to the dispersion of light. These effects are often used for artistic purposes or in decorative items.
- Environmental Monitoring: Dispersion can also be used to monitor environmental changes. For instance, changes in the dispersion patterns of sunlight can indicate the presence of pollutants in the atmosphere.
These applications show how the fundamental concept of light dispersion is applied in various fields, enhancing our scientific understanding and contributing to practical solutions in everyday life.
Also Read: Refraction Through A Prism
Questions
Q: What happens if you use a material other than glass for the prism?
When a material other than glass is used for a prism, the dispersion of light—that is, the separation of white light into its constituent colors—can vary significantly. This variation is primarily due to differences in the material’s optical density and refractive index, which are key factors in how much light bends when passing through the prism.
The optical density of a material is a measure of how much it slows down light. A material with a higher optical density will slow down light more, leading to a greater bending or refraction of the light rays. This means that materials with higher optical densities will generally cause more dispersion.
The refractive index is a number that describes how much a material can bend light. It varies with the wavelength or color of the light, a phenomenon known as dispersion. Materials with higher refractive indices will disperse light more than those with lower refractive indices. For example, a diamond has a higher refractive index than glass and thus will disperse light into a more vivid spectrum.
Different materials will produce different dispersion characteristics. For instance:
- Diamond: Known for its high dispersion, resulting in a very colorful spectrum.
- Flint Glass: Has a higher dispersion than standard crown glass, leading to a wider spectrum.
- Acrylic: Typically has a lower refractive index than glass so it would produce less dispersion.
The spectrum quality produced by a prism also depends on the material. Some materials may produce a sharper, more defined spectrum, while others might result in a more blurred or less distinct spectrum.
The choice of material for a prism is often based on the desired application. For broad-spectrum spectroscopy, where a wide range of wavelengths needs to be covered, materials that provide good dispersion without too much absorption are preferred.
Using different materials for prisms affects the degree and quality of light dispersion. The choice of material will depend on the specific needs of the application, such as the required precision of the spectral lines and the range of wavelengths to be analyzed.
Q: Can you explain the concept of critical angle in non-glass prisms?
The critical angle is the angle of incidence above which light is internally reflected within a material. When light travels from a denser medium to a rarer medium (like from water to air), there’s a specific angle of incidence at which the refracted ray of light skims the surface. This specific angle is known as the critical angle.
For non-glass prisms, such as those made of water, acrylic, or diamond, the critical angle will differ because each material has a unique refractive index. The refractive indices of the two media at the interface determine the critical angle.
The critical angle (θc) can be calculated using Snell’s Law, which relates the angle of incidence (θi) to the angle of refraction (θr). The formula for the critical angle is:
\(\displaystyle \theta_c = \sin^{-1}\left(\frac{n_r}{n_i}\right) \)
where (ni) is the refractive index of the denser medium (inside the prism) and (nr) is the refractive index of the rarer medium (outside the prism).
For example, if you have a prism made of water (with a refractive index of about 1.33) in air (with a refractive index of about 1.00), the critical angle can be calculated as:
\(\displaystyle \theta_c = \sin^{-1}\left(\frac{1.00}{1.33}\right) \)
which gives a critical angle of about 48.6 degrees. Any light hitting the water-air interface at an angle greater than 48.6 degrees will be internally reflected.
The critical angle is a fundamental concept in optics that depends on the refractive indices of the materials involved. It’s the angle beyond which light cannot pass through the interface but is instead reflected into the material, and it varies for different materials used in prisms.
Q: How does dispersion change with irregular-shaped prisms?
Dispersion of light in irregular-shaped prisms can be quite different from what we observe in regular triangular prisms. In a regular prism, the path of light is predictable, with light entering and exiting at specific angles. In an irregular-shaped prism, the angles can vary greatly, leading to a more complex path for the light as it travels through the prism.
The angle of refraction, which is the angle at which light bends when entering or exiting the prism, can differ significantly in irregular shapes. This means that the angle of deviation for each color of light could be less uniform compared to a triangular prism.
The spread of the spectrum can also be affected. In a triangular prism, the spectrum is usually linear and orderly. In an irregular-shaped prism, the spectrum could be spread out in a non-linear fashion, potentially creating a more scattered pattern of colors. Due to the varying angles and surfaces, some colors may overlap or mix, leading to a less distinct separation of the spectrum. This can result in a blending of colors rather than a clear division between them.
The intensity of the dispersed colors might also change. Some colors may appear brighter or more pronounced, while others could be less visible, depending on the shape of the prism and how it affects the light’s path. Irregular shapes can cause light to pass through varying thicknesses of the prism material, which can alter the refractive index experienced by different light rays. This can further influence the dispersion pattern.
A regular prism produces a predictable and orderly spectrum, an irregular-shaped prism can create a more complex and less predictable pattern of dispersed light. The exact nature of the dispersion will depend on the specific shape and material of the prism.
Q: How does the angle of deviation change with different prism shapes?
The angle of deviation changes with different prism shapes due to the varying angles at which light enters and exits the prism, and the path it takes through the material. Here’s how different shapes influence the angle of deviation:
- Triangular Prisms: For a triangular prism, which is the most common type used to demonstrate dispersion, the angle of deviation depends on the refractive index of the material and the prism’s apex angle. The apex angle is the angle between the two faces of the prism through which light enters and exits. A larger apex angle generally results in a larger angle of deviation because the light spends more time inside the prism, increasing the opportunity for refraction.
- Rectangular Prisms: Rectangular prisms can also cause deviation of light, but since the angles at which light enters and exits are usually 90 degrees, there is no dispersion, and the deviation is minimal. The light path is essentially parallel-shifted.
- Pentagonal Prisms: Pentagonal prisms have more faces, which means that light can undergo multiple refractions. This can lead to a complex path of light within the prism, potentially increasing the angle of deviation depending on the arrangement of the faces.
- Prism Material: The material of the prism also affects the angle of deviation. Different materials have different refractive indices, which means that the same shape of prism made from different materials will deviate light by different amounts.
- The angle of Incidence: The angle at which light enters the prism, known as the angle of incidence, also plays a significant role. If the light enters at a steeper angle relative to the prism’s surface, the angle of deviation will be larger.
So, the angle of deviation is influenced by the shape of the prism, the material’s refractive index, and the angle of incidence. Each of these factors can alter the path of light through the prism, resulting in different angles of deviation for different prism shapes
FAQs
What is the dispersion of white light by a glass prism and why does it occur?
Dispersion of white light by a glass prism occurs when white light passes through the prism and splits into its constituent colors. This happens because different colors (wavelengths) of light refract by different amounts as they pass through the prism. The variation in the refractive index for different wavelengths causes this separation, with violet light bending the most and red light bending the least.
What is the visible light spectrum, and what are its primary colors?
The visible light spectrum is the range of electromagnetic waves that are visible to the human eye, typically ranging from approximately 400 nm (violet) to 700 nm (red). The primary colors in the visible spectrum, in order of increasing wavelength, are violet, indigo, blue, green, yellow, orange, and red.
What was Newton’s prism experiment and what did it demonstrate about light?
Newton’s prism experiment involved passing a beam of sunlight through a glass prism, which resulted in the dispersion of light into a spectrum of colors. He further demonstrated that by passing the dispersed light through a second prism, the colors could be recombined to form white light again. This experiment proved that white light is composed of multiple colors and that prisms can separate and recombine these colors through refraction.
How are rainbows formed, and what role does dispersion play in their formation?
Rainbows are formed when sunlight passes through raindrops in the atmosphere. Each raindrop acts like a prism, refracting and internally reflecting the light, which then exits the drop and disperses into its constituent colors. The dispersion of light within the raindrop separates the colors, creating the circular spectrum observed in a rainbow.
What causes the scattering of light and why is the sky blue?
The scattering of light is caused by the interaction of light with small particles in the atmosphere. Shorter wavelengths of light (blue and violet) are scattered more than longer wavelengths (red and orange). Although violet light is scattered more, our eyes are more sensitive to blue light, and some violet light is absorbed by the upper atmosphere, making the sky appear blue during the day.
What is the phenomenon of a double rainbow and how does it occur?
A double rainbow occurs when light is reflected twice inside raindrops before emerging. The second reflection causes the formation of a secondary rainbow with colors reversed and appearing outside the primary rainbow. This secondary bow is usually fainter due to the extra reflection reducing the intensity of the light.
How does the scattering of light explain the reddish color of the sunset?
The reddish color of the sunset is explained by Rayleigh scattering. During sunset, the sun’s light has to pass through a greater thickness of the Earth’s atmosphere, which scatters shorter wavelengths (blue and violet) out of the direct path. This leaves the longer wavelengths (red and orange) to dominate the sky’s color, creating a reddish appearance at sunset.