The Law of Inertia, often referred to as Newton’s First Law of Motion, has a fascinating history that spans several centuries and involves a series of discoveries and refinements. Initially, the Greek philosopher Aristotle believed that an external force was necessary to maintain an object in motion. This view was widely accepted until the Renaissance period.
The first significant challenge to Aristotle’s view came from Galileo Galilei. Through his experiments, Galileo observed that objects tend to keep moving unless a force, such as friction, stops them. He conducted experiments with balls rolling down inclined planes and deduced that a body in motion would remain in motion unless acted upon by a force.
Following Galileo, René Descartes generalized the concept of inertia. He proposed that this tendency to resist changes in motion applied not just to objects on Earth but to all matter in the universe.
Finally, Sir Isaac Newton formulated the Law of Inertia as we know it today. Newton’s First Law states that an object at rest stays at rest and an object in motion stays in motion with the same speed and in the same direction unless acted upon by an unbalanced force. This law laid the foundation for classical mechanics.
The Principle of Inertia: The principle of inertia was crucial for understanding the motion of celestial bodies. It helped explain why we do not sense the Earth’s motion as it spins on its axis and orbits the Sun. The principle suggests that since we are in motion together with Earth, and our natural tendency is to retain that motion, Earth appears to us to be at rest.
Interestingly, the dynamical aspect of the Law of Inertia has roots in the medieval impetus tradition, which originated from the sixth-century Neoplatonist John Philoponus and was later developed through Arabic commentaries. This tradition explored the cause of a projectile’s continued motion once it had separated from the projector.
What Is the Law of Inertia?
The Law of Inertia, also known as Newton’s First Law of Motion, states that an object will remain at rest or in uniform motion in a straight line unless acted upon by an external force. This means that objects resist changes to their state of motion.
Imagine you’re sitting in a car at a stoplight. When the light turns green, and the car suddenly starts, you feel pushed back into your seat. Conversely, when the car comes to a stop, you lurch forward. This experience is a common everyday encounter with the Law of Inertia.
Newton’s First Law of Motion, tells us that there’s a natural tendency of objects to resist changes in their state of motion. In simpler terms, things like to keep doing what they’re currently doing.
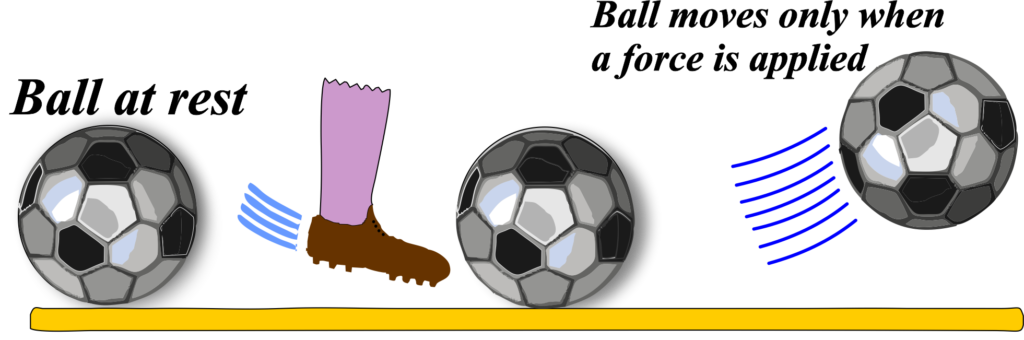
At Rest and In Motion
- At Rest: If an object is at rest (like a ball on the ground), it will stay at rest until something or someone comes along and kicks it or picks it up.
- In Motion: If an object is moving (like a skateboard rolling down the street), it will keep moving in the same direction and at the same speed, unless something stops it or changes its direction (like grabbing it or hitting a rock).
This resistance to change doesn’t come from an actual force but from the object’s inertia. It’s like an object’s stubbornness to change what it’s doing unless it has to.
Understanding inertia is crucial because it’s not just about objects at rest or in motion; it’s about understanding the fundamental behavior of everything around us. Whether it’s a car, a planet, or a galaxy, the Law of Inertia governs how they move.
Examples:
- When you’re riding a bicycle and suddenly stop pedaling, the bike doesn’t stop immediately; it keeps going forward. That’s inertia.
- Earth keeps orbiting the sun in a nearly circular path because of inertia, combined with the gravitational pull of the sun.
Galileo’s Free Fall Experiment
Galileo hypothesized that objects gain velocity at a constant rate when falling. Since he couldn’t observe free-falling objects directly, he conducted experiments with balls rolling down inclined planes to slow down the motion and study it.
Before Galileo, people believed Aristotle’s theory that heavier objects fall faster than lighter ones. But Galileo wasn’t convinced. He thought that all objects should fall at the same rate, regardless of their weight.
The Tower of Pisa: Legend has it that Galileo climbed to the top of the Leaning Tower of Pisa and dropped two spheres of different masses. To the surprise of onlookers, both spheres hit the ground at the same time. This simple yet powerful demonstration challenged centuries of misconceptions.
Galileo knew that to study falling objects, he needed to slow down their descent. So, he used inclined planes—ramps. By rolling balls down these ramps, he could carefully observe and measure their motion, which would be too fast to see during free fall.
Through his experiments, Galileo discovered that all objects accelerate at the same rate when they’re falling. This rate is now known as the acceleration due to gravity, (g), which is approximately ( 9.81 m/s2 ) on Earth.
Galileo also found that the distance (d) a falling object travels is directly proportional to the square of the time (t) it takes to fall. Mathematically, this relationship can be expressed as:
\(\displaystyle d = \frac{1}{2} g t^2 \)
Galileo’s findings were revolutionary. They contradicted the long-held belief that heavier objects fall faster and laid the groundwork for Newton’s laws of motion. Galileo’s work showed the importance of experimentation and observation in science, changing our understanding of the physical world forever.
Also Read: Motion in a Plane
Experiment on Inclined Plane
Galileo’s inclined plane experiment showed that a ball rolls down a slope with an acceleration that is constant. This supported his hypothesis about objects gaining speed uniformly when falling.
Imagine you have a ramp and a ball. When you place the ball at the top of the ramp and let go, it rolls down. This setup is what we call an inclined plane—a flat surface tilted at an angle.
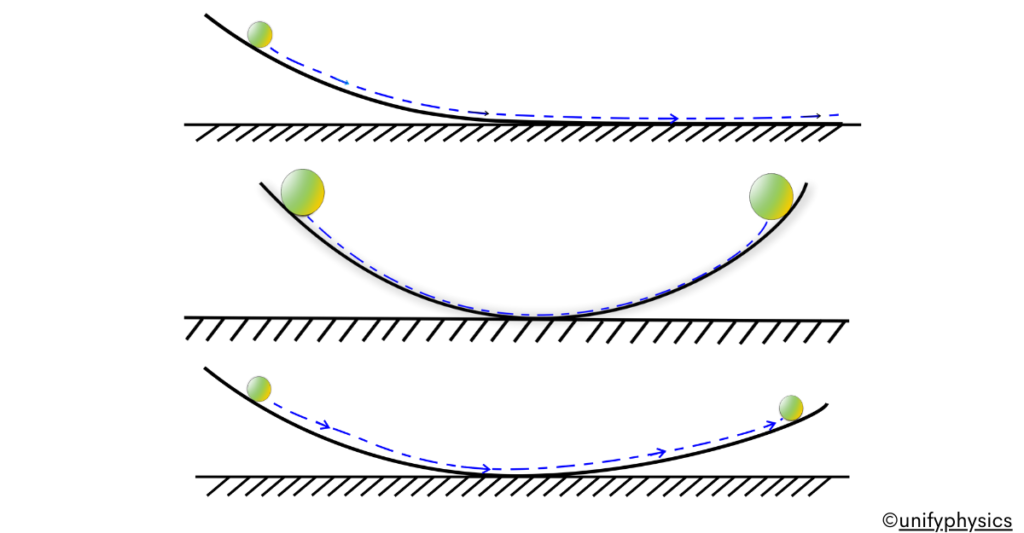
Rolling a ball down one slope and up another. If both slopes are smooth, the ball will end up almost as high as where it started, though a little lower. In a perfect world without friction, it would end up exactly where it started.
Now, if you make the second slope less steep, the ball still reaches the same height, but it travels a longer distance. If the second slope is completely flat, the ball keeps rolling forever because there’s no friction to stop it.
In real life, friction eventually slows the ball down on the flat surface. But if there was no friction at all, the ball would keep rolling at the same speed forever.
This helped Galileo understand something new about motion: Being still and moving at a steady speed are the same thing.
Galileo used this simple setup to study motion. He wanted to understand how objects speed up when they fall and how the force of gravity affects them.
Observation: When you release the ball at the top, it starts rolling down slowly and then picks up speed as it goes further down the ramp. This change in speed is called acceleration.
Galileo rolled balls down ramps with different slopes. He measured how far the ball traveled over time and found that the ball’s speed increased at a constant rate. This was groundbreaking because it showed that acceleration is consistent, not random.
Two main forces are acting on the ball:
- Gravity: Pulling the ball down the slope.
- Normal Force: Pushing perpendicular to the surface of the ramp, supporting the ball.
Gravity’s force can be split into two components:
- Parallel to the Ramp: This part makes the ball roll down.
- Perpendicular to the Ramp: This part is balanced by the normal force.
Using some trigonometry, Galileo could calculate the component of gravity pulling the ball down the ramp. This allowed him to predict the ball’s motion.
This experiment was crucial because it helped Galileo develop the concept of inertia and laid the foundation for Newton’s laws of motion. It showed that the natural world follows predictable laws that can be expressed mathematically.
Moment of Inertia
The moment of inertia measures an object’s resistance to changes in its rotational motion. It depends on the object’s mass and the distribution of that mass relative to the axis of rotation.
Think of the Moment of Inertia as the rotational equivalent of mass in linear motion. Just like mass determines how much an object resists being moved, Moment of Inertia determines how much an object resists being rotated.
Imagine you’re trying to push a merry-go-round. If it’s empty, it’s relatively easy to spin. But if it’s full of people, it’s much harder. That resistance to change in rotational motion is what we call the Moment of Inertia.
Mathematically, the Moment of Inertia (I) is calculated by taking the sum of all the masses (mi) of the particles that make up an object, each multiplied by the square of its distance (ri) from the axis of rotation:
\(\displaystyle I = \sum m_i r_i^2 \)
You might wonder why we square the distance. It’s because the further away a mass is from the axis, the more it ‘counts’ towards the Moment of Inertia. This is why pushing a merry-go-round is harder when people are sitting on the edge rather than close to the center.
Different shapes have different Moments of Inertia because their mass is distributed differently. For example, a solid disk and a hoop might have the same mass, but the hoop has a larger Moment of Inertia because more of its mass is farther from the center.
Examples:
- Figure Skaters: When a figure skater pulls their arms in, they spin faster because they’re decreasing their Moment of Inertia.
- Door Hinges: Doors have hinges placed at one side rather than the middle because it changes the Moment of Inertia, making the door easier to swing open.
Types of Inertia
Inertia of Rest
Imagine you’re at a bus stop, and a bus comes to a halt in front of you. It’s not going anywhere until the driver decides to hit the gas pedal. This is an everyday example of Inertia of Rest.
The inertia of Rest is the tendency of an object to remain in its state of rest unless an external force acts on it. It’s like saying, “If I’m sitting comfortably, I’m not moving unless I have a good reason to.”
Every object has mass, and this mass doesn’t like to change what it’s doing. If it’s sitting still, it wants to keep sitting still. This ‘laziness’ is a fundamental property of matter.
Understanding Inertia of Rest helps us predict how objects will behave. For example:
- A book will stay on your desk until you pick it up.
- A parked car won’t move until someone starts the engine.
You can demonstrate the Inertia of Rest with a simple experiment. Place a coin on top of a card that’s resting on a cup. Flick the card quickly, and the coin will drop into the cup. The card moved because you applied a force, but the coin didn’t move with the card because of its Inertia of Rest.
Inertia of Direction
Imagine you’re in a car that’s turning a corner. You feel a force pushing you towards the outside of the turn. This is a common experience of Inertia of Direction.
Inertia of Direction is the tendency of an object to continue moving in the same direction unless an external force causes it to change that direction. It’s like saying, “I’m going straight, and I’m not changing course unless something makes me.”
This type of inertia is all about maintaining the current path of motion. An object moving in a straight line wants to keep moving in that line. It doesn’t want to turn or change direction on its own.
Understanding Inertia of Direction helps us predict how objects will move when they encounter forces that try to change their direction. For example:
- A soccer ball kicked in a straight line will not curve unless acted upon by a player, the wind, or another force.
- Planets orbiting the sun continue in a curved path because the gravitational pull of the sun constantly changes their direction.
You can demonstrate Inertia of Direction with a simple experiment. Roll a ball in a straight line on a smooth surface. It will continue in that straight line until it hits something that changes its direction, like a wall or your foot.
Inertia of Motion
Inertia of Motion is the tendency of an object to continue moving at a constant velocity unless acted upon by an external force. It’s like when you’re riding a bicycle; once you get going, you can coast without pedaling because of inertia.
This type of inertia is all about maintaining the current speed and direction. An object in motion wants to keep moving in the same way – straight and steady.
Examples:
- Space Probes: Once they’re far from Earth, space probes need very little energy to keep moving because there’s almost no friction in space to stop them.
- Ice Skating: When you skate across the ice and stop pushing with your feet, you still glide forward due to inertia.
Understanding Inertia of Motion helps us predict how objects will move when they encounter forces that try to change their speed or direction. For example:
- A rolling ball will continue rolling until friction slows it down and stops it.
- A car will keep moving forward until the brakes are applied, which exert a force to change its state of motion.
You can demonstrate Inertia of Motion by rolling a ball on a smooth surface and observing how it moves. The smoother the surface, the less friction there is, and the longer the ball will keep rolling.
If we want to describe this mathematically, we can say that an object with mass (m) moving at velocity (v) will have a momentum (p), which is the product of mass and velocity:
\(\displaystyle p = m \times v \)
Inertia of Motion is a fundamental concept that explains why objects don’t just stop on their own. It’s a principle that plays a crucial role in everything from sports to vehicle safety to the movement of celestial bodies.
Limitations of Law of Inertia
The Law of Inertia applies only to ideal conditions without external forces like friction or air resistance. In the real world, these forces can affect motion.
- The Law of Inertia works perfectly in an ideal, frictionless world. However, in the real world, forces like friction and air resistance are always at play, affecting the motion of objects.
- Inertia tells us that an object will not change its motion unless an external force acts on it. But this doesn’t mean that an object will continue to move forever once it’s in motion. For example, a ball rolling on the ground will eventually stop because of friction.
- While the Law of Inertia applies to objects in space, on Earth, gravity is a constant force that affects all objects. Whether an object is at rest or in motion, gravity is always pulling it downward.
- The Law of Inertia is straightforward when dealing with straight-line motion. But when objects move in complex ways, like in circular motion or oscillation, other principles and forces come into play, and the Law of Inertia alone cannot describe the motion fully.
- At the quantum level (very small scales) or speeds close to the speed of light, classical physics, including the Law of Inertia, doesn’t hold. Quantum mechanics and the theory of relativity are needed to explain motion in these realms.
- Inertia assumes that objects are not capable of changing their state of motion. But living things, like humans or animals, can move on their own, which is not covered by the Law of Inertia.
Law of Inertia Examples
Sudden Start of a Vehicle: When a bus or car starts suddenly, passengers feel a push backward. This happens because the lower part of the body in contact with the vehicle moves forward, but the upper part tends to remain at rest due to inertia.
Jerk in an Elevator: If you’re in an elevator and it starts or stops suddenly, you feel a jerk. This is because your body resists the sudden change in motion – whether it’s starting to move (inertia of rest) or coming to a stop (inertia of motion).
Dusting a Carpet: When you beat a carpet with a stick, the carpet moves quickly, but the dust particles tend to stay in place due to their inertia. As a result, they separate from the carpet and come out.
Braking Suddenly: When you’re in a moving vehicle that brakes suddenly, you lurch forward. Your body wants to keep moving at the same speed and direction (inertia of motion), but the seatbelt or another object provides the external force to stop you.
Artificial Satellites: Artificial satellites keep moving in their orbits due to inertia. Once they are set in motion, they tend to stay in motion, following a path around the Earth unless acted upon by external forces like atmospheric drag.
Shaking a Tree: When you shake a tree, the branches move, but the fruits or leaves tend to stay at rest. Due to their inertia, they eventually break free from the tree and fall down.
FAQs
How does the law of inertia apply to everyday situations?
The law of inertia, often stated as “an object in motion stays in motion, and an object at rest stays at rest unless acted upon by an external force,” governs various scenarios. For instance, when a car abruptly stops, passengers lurch forward due to their inertia, resisting the change in motion until a force (like a seatbelt) acts on them.
Can inertia be perceived in space where there’s no gravity?
Absolutely. In space, where gravitational forces are negligible, objects still exhibit inertia. Astronauts experience this when they push off from surfaces; they continue moving until another force, like friction with a wall or the pull of a tether, acts upon them.
How does inertia differ from momentum?
While both concepts deal with motion, inertia refers to an object’s tendency to maintain its state of motion (or rest), whereas momentum involves the quantity of motion an object possesses. Inertia is a property of matter, while momentum depends on both the object’s mass and velocity.
Is inertia affected by an object’s mass?
Yes, inertia is directly related to mass. Objects with greater mass exhibit greater inertia, meaning they are more resistant to changes in their state of motion. This relationship is evident in Newton’s second law, which states that force equals mass times acceleration.
Can inertia be overcome?
Inertia can indeed be overcome by applying an external force. Any force exerted on an object can alter its state of motion or rest, as per Newton’s first law. This principle underlies the functioning of brakes in vehicles, which apply a force to counteract the inertia of moving objects.
Does inertia apply only to linear motion, or does it extend to rotational motion as well?
Inertia applies to both linear and rotational motion. Just as an object in linear motion tends to maintain its velocity, a rotating object tends to maintain its rotational speed and direction unless acted upon by an external torque. This principle is crucial in understanding the behavior of rotating bodies.
How does inertia contribute to the safety features in modern vehicles?
Inertia plays a vital role in the design of safety features like airbags and crumple zones. These features are engineered to mitigate the effects of sudden deceleration by increasing the time over which the change in momentum occurs. By doing so, they reduce the force experienced by occupants, minimizing injuries during collisions.