Ancient philosophers, such as Thales of Miletus around 550 BCE, had inklings about the conservation of some underlying substance from which everything is made.
However, their theories didn’t directly align with our modern understanding of energy. For example, Thales believed this fundamental substance was water.
Empedocles (490–430 BCE) proposed a universal system composed of four elements: earth, air, water, and fire. He asserted that “nothing comes to be or perishes” within this system; instead, the elements undergo continual rearrangement.
In 1842, German physicist Julius Mayer was the first to state the law of the conservation of energy formally. Mayer experimentally determined the mechanical equivalent of heat by studying the heat that evolved during the compression of a gas. Interestingly, he didn’t fully appreciate that heat could be explained in terms of kinetic theory.
French mathematician and philosopher Émilie du Châtelet proposed and tested the energy conservation law in the 18th century. Her work contributed significantly to our understanding of energy conservation.
Heat
Heat is a form of energy that is transferred between systems or objects with different temperatures (thermal energy). It flows from a hotter object to a cooler one and can be measured in joules, calories, or BTUs.
Heat is a form of energy that is transferred between two objects or systems due to a temperature difference. It is not something that is contained within an object, but rather it is the energy in transit during the process of temperature equalization.
Examples:
Touching a Hot Cup of Coffee:
- When you touch a hot cup of coffee, you feel heat because energy is being transferred from the cup to your hand.
- This transfer continues until the temperature of your hand and the cup are the same.
Sunbathing:
- While sunbathing, your body absorbs heat from the sun, increasing your body temperature.
- The heat you feel is the transfer of energy from the sun’s rays to your skin.
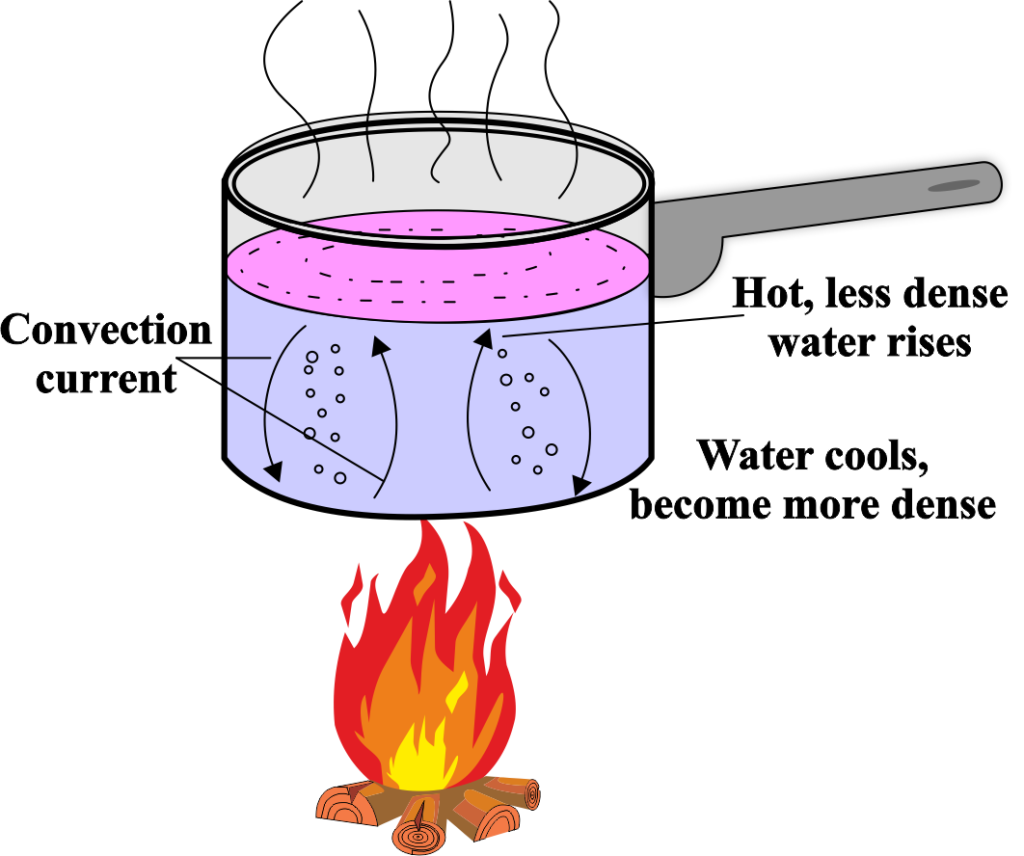
Heat can move in three ways: conduction, convection, and radiation. Conduction is the transfer of heat through a solid material, like a metal spoon in a pot of boiling water.
Convection occurs in fluids (liquids and gases) where warmer areas of a fluid rise and cooler areas sink, creating a cycle.
Radiation is the transfer of heat through electromagnetic waves, like the heat from the sun.
Unit:
- The unit of heat in the International System of Units (SI) is the joule (J).
- However, heat is also sometimes measured in calories (cal) or British Thermal Units (BTU).
It’s important to distinguish between heat and temperature. Temperature is a measure of the average kinetic energy of the particles in a substance. Heat refers to the total energy transferred due to temperature differences.
Heat plays a crucial role in phase changes, such as melting, boiling, or freezing. During these changes, heat is either absorbed or released by the substance, but the temperature remains constant.
Chemical Energy
Chemical energy is the energy stored in the bonds of chemical compounds, such as molecules and atoms. It’s like the energy you have stored in a battery; it’s not doing anything right now, but once you use the battery, that energy is released to power your device.
Think of chemical energy as potential energy. It’s waiting to be released, much like a stretched rubber band. When a chemical reaction occurs, this energy is released, often producing heat as a by-product. This is what happens when you light a match; the chemical energy in the match head is released as heat and light.
Chemical Energy in Everyday Life:
- The food we eat contains chemical energy. When we digest food, our bodies break down the food molecules, releasing the energy we need to live and move.
- Batteries store chemical energy that is transformed into electrical energy to power our electronic devices.
Conservation of Chemical Energy:
- In a closed system, the total chemical energy before and after a reaction remains constant.
- However, the form of energy may change. For example, when gasoline burns in a car engine, chemical energy is converted into mechanical energy to move the car.
Types of Chemical Reactions:
Exothermic Reactions
Exothermic reactions are chemical reactions that release energy by light or heat. It’s the opposite of an endothermic reaction, which requires energy to proceed.
During an exothermic reaction, the energy needed to break the bonds in the reactants is less than the energy released when new bonds form in the products. This excess energy is given off as heat, and sometimes light, which is why exothermic reactions often feel hot or are luminous.
Examples:

Combustion:
- The burning of wood, coal, or gasoline is a classic example of an exothermic reaction.
- When these substances combust, they release heat energy that can be used to warm houses or power engines.
Respiration:
- In our bodies, cells perform respiration, which is an exothermic process.
- Glucose combines with oxygen to produce carbon dioxide, water, and energy in the form of heat.
Exothermic reactions are crucial for many everyday processes, from heating our homes to powering our vehicles. They also play a significant role in industrial processes, such as the production of steel and cement.
The substances involved in exothermic reactions contain chemical potential energy. When a reaction occurs, this potential energy is converted into kinetic energy (heat).
In exothermic reactions, the law of conservation of energy still applies. The energy isn’t lost; it’s simply transformed from chemical potential energy into thermal energy.
A simple classroom experiment to demonstrate an exothermic reaction is the reaction between vinegar and baking soda. When combined, they react to form carbon dioxide gas and water, and the mixture becomes warmer, indicating an exothermic reaction.
Endothermic reactions
Endothermic reactions absorb energy from their surroundings. Photosynthesis in plants is a common example, where energy from sunlight is absorbed to convert carbon dioxide and water into glucose and oxygen.
Endothermic reactions are chemical processes that absorb energy from their surroundings, typically in the form of heat. This is the energy that’s needed to break the bonds in the reactants, and it’s greater than the energy released when new bonds form in the products.
These reactions often result in a temperature decrease in the surrounding environment because they absorb heat. The energy absorbed is usually necessary for the reaction to occur, providing what’s known as the activation energy.
Examples:

Photosynthesis:
- In photosynthesis, plants absorb sunlight to convert carbon dioxide and water into glucose and oxygen.
- This process stores solar energy as chemical energy in glucose.
Dissolving Certain Salts in Water:
- When salts like ammonium chloride are dissolved in water, the solution becomes colder.
- This is because the salt absorbs heat from the water to break its ionic bonds and dissolve.
The enthalpy change (∆H) in an endothermic reaction is positive, indicating that the system gains heat from the surroundings. More energy is required to break the initial bonds than is released during the formation of new bonds.
A simple way to demonstrate an endothermic reaction is to mix barium hydroxide with ammonium thiocyanate. As the chemicals react, they draw heat from the surroundings, causing the beaker to feel cold and even freeze on the tabletop.
Electrical Energy
Electrical energy is the energy that’s associated with electric charges and their movements. Electrical energy is the movement of electrons through a conductor. It’s used to power everything from light bulbs to computers and is easily converted from other energy forms like mechanical or solar energy.
It can come from both electric potential energy (which is energy stored due to the position of charges) and kinetic energy (which is energy due to the motion of charges).
Electrical energy is produced when charged particles, like electrons, move through a conductor, like a wire. This movement, known as electric current, is what powers our electronic devices.
When a voltage is applied across a conductor, it creates an electric field that pushes the electrons, causing them to flow. This flow of electrons is what we harness as electrical energy.
The amount of electrical energy used can be calculated using the formula:
\(\displaystyle E = P \times t \)
where (E) is the energy in joules, (P) is the power in watts, and (t) is the time in seconds.
The basic unit of electrical energy is the joule (J), which is also known as a watt-second. For larger quantities, we use kilowatt-hours (kWh), which is the commercial unit of electrical energy.
Examples: 1) When you turn on a light bulb, electrical energy is converted into light and heat energy. 2) Plugging in your phone uses electrical energy to recharge the battery, storing the energy for later use.
According to the law of conservation of energy, electrical energy can be transformed into other forms of energy but cannot be created or destroyed. In a circuit, electrical energy can be converted into heat, light, mechanical energy, and more.
The Equivalence of Mass and Energy
Albert Einstein’s famous equation \(\displaystyle E=mc^2\) shows that mass and energy are interchangeable. This means that energy has mass, and mass contains energy. The equation tells us that a small amount of mass can be converted into a large amount of energy, which is the principle behind nuclear power.
In other words, mass possesses a certain amount of energy simply by virtue of its existence. This concept revolutionized our understanding of the universe, revealing that matter and energy are fundamentally interconnected.
To understand this concept, consider the process of nuclear reactions, such as those that occur in the core of stars or in nuclear power plants. In these reactions, a small amount of mass is converted into a large amount of energy. This is because the mass lost during the reaction is converted into energy according to Einstein’s equation.
Conversely, energy can be converted into mass under certain conditions. For example, in particle accelerators, high-energy collisions can create new particles with mass from the kinetic energy of the colliding particles.
Imagine you have a tiny marble that weighs 20 grams. According to Einstein’s equation, if you could convert that entire marble into energy, it would release an amount of energy equivalent to a large hydrogen bomb.
But don’t worry, marbles aren’t dangerous because converting mass into energy is not something that happens easily.
One way to convert all the mass of an object into energy is through a process called matter-antimatter annihilation. When a particle meets its antiparticle, they can annihilate each other, releasing energy in the form of electromagnetic waves.
This concept is crucial for understanding how the sun shines (through nuclear fusion) and how nuclear reactors work (through fission). It also explains why we can’t create perpetual motion machines because energy and mass are conserved; they can’t be created from nothing.
Nuclear Energy
Nuclear energy is the energy held in the nucleus, the core of an atom, where protons and neutrons reside. Atoms are tiny particles that make up every material in the universe, and the nucleus is like the sun at the center of the solar system: small but incredibly dense and full of energy.
Nuclear energy comes from splitting (fission) or combining (fusion) atomic nuclei. In nuclear reactors, fission is used to produce heat, which is then converted into electrical energy.
Fission is when the nucleus of an atom splits into two or more smaller nuclei, along with a few neutrons and a large amount of energy.

Nuclear Fission: In nuclear power plants, fission is used to produce energy. A heavy element, like uranium or plutonium, is bombarded with neutrons, causing it to split and release energy. This energy is used to heat water, produce steam, and drive turbines to generate electricity.
Fusion is when two light nuclei join together to form a heavier nucleus, which also releases energy.
Nuclear Fusion: Fusion is the process that powers the sun and other stars. It involves combining small nuclei, like hydrogen, at very high temperatures and pressures to form helium and release energy. Fusion has the potential to be a cleaner and more abundant source of energy than fission, but it’s currently not as well-developed for practical use.

Uses:
- Nuclear energy is a powerful source of energy that can generate large amounts of electricity without emitting greenhouse gases during operation.
- It’s considered a more “climate-friendly” alternative to fossil fuels, as it can produce electricity continuously, unlike some renewable sources that depend on weather conditions.
Safety and Challenges:
- While nuclear energy is efficient, there are concerns about radioactive waste, which remains hazardous for thousands of years, and the potential for catastrophic accidents, like Chernobyl or Fukushima.
- The future of nuclear energy may involve advanced reactors with higher safety standards and methods to deal with waste more effectively.
Also Read: Conservation of Mechanical Energy
The Principle of Conservation of Energy
This principle states that the total energy in an isolated system remains constant. It may change form, from kinetic energy to potential energy, but the total amount of energy remains the same. This is a fundamental concept in physics and underlies all energy transformations.
The law of conservation of energy is a fundamental concept in physics. It states that within a closed system, energy can change form, but the total amount of energy remains constant.
In simpler terms: Energy cannot be created or destroyed; it can only be transformed from one form to another. If we consider all forms of energy, their total sum remains unchanged.
Closed Systems:
- The principle applies to isolated systems that do not exchange energy with their surroundings.
- For example, if we look at the entire universe as an isolated system, the total energy within it remains constant.
Energy exists in various forms: kinetic, potential, thermal, chemical, nuclear, and more. While energy can change from one form to another, the total energy remains the same.
The total energy of a system can be expressed as:
\(\displaystyle U_{T} = U_{i }+ W + Q \)
- (UT) represents the total energy.
- (Ui) is the initial energy of the system.
- (W) represents work done by or on the system.
- (Q) represents heat added or removed from the system.
Imagine a fruit falling from a tree. Initially, it has potential energy due to its height. As it falls, the potential energy decreases, and kinetic energy increases. The total energy (potential + kinetic) remains constant.
When applying conservation of energy, we define a system that includes the objects of interest and their interactions. We draw a boundary around what we care about and exclude other interactions (the environment).
Conservation of energy helps us understand everything from simple pendulums to complex machinery.It’s essential for predicting behavior in mechanical systems, electrical circuits, and more.
Solved Examples
Example 1:A car of mass (1000 kg) is moving with a speed of (20 m/s). Calculate its kinetic energy.
Solution: Given; Mass of the car, (m = 1000 kg) ; Speed of the car, (v = 20 m/s)
Kinetic energy (K) is given by the formula:
\(\displaystyle K = \frac{1}{2} m v^2 \)
Substitute the given values:
\(\displaystyle K = \frac{1}{2} \times 1000 \times (20)^2 \)
\(\displaystyle K = \frac{1}{2} \times 1000 \times 400 \)
\(\displaystyle K = 200000 \, \text{J} \)
Therefore, the kinetic energy of the car is (200000 J).
Example 2: A book of mass (2 kg) is placed on a shelf (3 m) above the ground. Calculate its gravitational potential energy with respect to the ground.
Solution: Given; Mass of the book, (m = 2 kg) ; Height of the shelf, (h = 3 m) ; Acceleration due to gravity, (g = 9.8 m/s2) Gravitational potential energy (U) is given by the formula:
\(\displaystyle U = mgh \)
Substitute the given values:
\(\displaystyle U = 2 \times 9.8 \times 3 \)
\(\displaystyle U = 58.8 \, \text{J} \)
Therefore, the gravitational potential energy of the book is (58.8 J).
Example 3: A cup of water initially at (20∘C) is heated until it reaches (80∘C). Calculate the increase in thermal energy of the water. Assume the specific heat capacity of water is (4200 J/kg∘C).
Solution: Given; Initial temperature, (Ti = 20∘C) ; Final temperature, (Tf = 80∘C) ; Specific heat capacity of water, (c = 4200 J/kg∘C) ; Mass of water, (m = 1 kg (for simplicity).
The increase in thermal energy (Q) is given by the formula:
\(\displaystyle Q = mc \Delta T \)
Where (∆T) is the change in temperature:
\(\displaystyle \Delta T = T_f – T_i \)
\(\displaystyle \Delta T = 80 – 20 \)
\(\displaystyle \Delta T = 60^\circ \text{C} \)
Substitute the given values:
\(\displaystyle Q = 1 \times 4200 \times 60 \)
\(\displaystyle Q = 252000 \, \text{J} \)
Therefore, the increase in thermal energy of the water is (252000 J).
Example 4: A battery with a capacity of (20000 J) is used to power a device. Calculate the amount of chemical energy stored in the battery.
Solution: Given; Battery capacity, (E = 20000 J)
The chemical energy stored in the battery is equal to its capacity:
\(\displaystyle \text{Chemical energy} = 20000 \, \text{J} \)
Therefore, the amount of chemical energy stored in the battery is (20000 J).
Example 5: A nuclear power plant generates (1 GW) of power continuously. Calculate the energy generated by the power plant in one hour.
Solution: Given; Power generated by the power plant, (P = 1 GW = 1 × 109 W) ; Time, (t = 1 hour = 3600 s)
Energy generated (E) is given by the formula:
\(\displaystyle E = Pt \)
Substitute the given values:
\(\displaystyle E = 1 \times 10^9 \times 3600 \)
\(\displaystyle E = 3.6 \times 10^{12} \, \text{J} \)
Therefore, the energy generated by the power plant in one hour is (3.6 ×1012 J).
Example 6: A car with a mass of (1000 kg) is moving at a speed of (10 m/s). Calculate its total energy and express it in terms of kinetic and potential energy.
Solution: Given; Mass of the car, (m = 1000 kg) ; Speed of the car, (v = 10 m/s)
The total energy (Etotal) of the car is the sum of its kinetic energy (K) and potential energy (U):
\(\displaystyle E_{\text{total}} = K + U \)
From Example 1, we know:
\(\displaystyle K = 50000 \, \text{J} \)
From Example 2, if we assume the car is on the ground, (U = 0 J) (since its height above the ground is zero).
Therefore:
\(\displaystyle E_{\text{total}} = 50000 + 0 = 50000 \, \text{J} \)
So, the total energy of the car is (50000 J), and it’s purely kinetic energy since there is no potential energy associated with it being on the ground.
FAQs
What are the various forms of energy, and how do they differ from each other?
There are several forms of energy, including kinetic energy (energy of motion), potential energy (energy due to position or configuration), thermal energy (energy associated with the temperature of an object), chemical energy (energy stored in chemical bonds), and nuclear energy (energy stored in the nucleus of atoms). These forms of energy differ based on their sources, manifestations, and ways in which they can be transformed from one form to another.
Can you explain how kinetic energy is related to an object’s mass and velocity?
Kinetic energy is directly proportional to both the mass and the square of the velocity of an object. The formula for kinetic energy is ( KE = \frac{1}{2}mv^2 ). This relationship means that an object with a greater mass or higher velocity possesses more kinetic energy.
How does potential energy differ from kinetic energy, and can it be negative?
Potential energy is energy associated with the position or configuration of an object, while kinetic energy is energy associated with its motion. Unlike kinetic energy, potential energy can be negative depending on the reference point chosen. For example, gravitational potential energy is negative when the reference point is set at a higher position than the object’s current position.
What role does thermal energy play in everyday life, and how is it related to the motion of particles?
Thermal energy is a form of internal energy associated with the temperature of an object. It manifests as the collective kinetic energy of the particles composing the object. In everyday life, thermal energy is responsible for phenomena like heating, cooling, and phase changes (e.g., melting, boiling). It reflects the motion and collisions of particles within a substance.
How is chemical energy stored in molecules, and how is it released during chemical reactions?
Chemical energy is stored in the bonds between atoms within molecules. These bonds store potential energy, which can be released during chemical reactions when new bonds are formed or existing bonds are broken. For example, the combustion of gasoline in a car engine releases chemical energy stored in the gasoline molecules, producing heat and kinetic energy.
What is nuclear energy, and how is it harnessed for power generation?
Nuclear energy is the energy stored in the nucleus of atoms, particularly during nuclear reactions such as fission or fusion. In nuclear fission, the nucleus of an atom is split into smaller nuclei, releasing a large amount of energy. In nuclear fusion, nuclei combine to form larger nuclei, also releasing energy. This energy is harnessed for power generation in nuclear power plants.
What is the Law of Conservation of Energy, and how does it apply to various forms of energy?
The Law of Conservation of Energy states that energy cannot be created or destroyed; it can only be transformed from one form to another or transferred from one object to another. This law applies to all forms of energy, including kinetic, potential, thermal, chemical, and nuclear energy. It ensures that the total energy of a closed system remains constant over time, even as energy is exchanged between different forms within the system.
Can you provide an example illustrating the Law of Conservation of Energy in action?
Consider a pendulum swinging back and forth. As the pendulum swings, it changes kinetic and potential energy. At the highest point of its swing, it has maximum potential energy and minimum kinetic energy, while at the lowest point, it has maximum kinetic energy and minimum potential energy. Despite these changes, the total mechanical energy (kinetic plus potential) remains constant, demonstrating the Law of Conservation of Energy.