An electric cell is a device that converts chemical energy into electrical energy by using electrodes and an electrolyte. An electrode is a conductor that participates in a chemical reaction with the electrolyte, which is a substance that can conduct electricity by producing ions. An electric cell has two electrodes: a positive electrode called the cathode and a negative electrode called the anode. When the electrodes are connected to an external circuit, a potential difference is created between them, which drives the flow of electrons from the anode to the cathode. This is called an electric current. The chemical reaction between the electrodes and the electrolyte also produces new substances, which gradually reduce the potential difference and the electric current. This is why electric cells eventually run out of energy and need to be replaced or recharged.
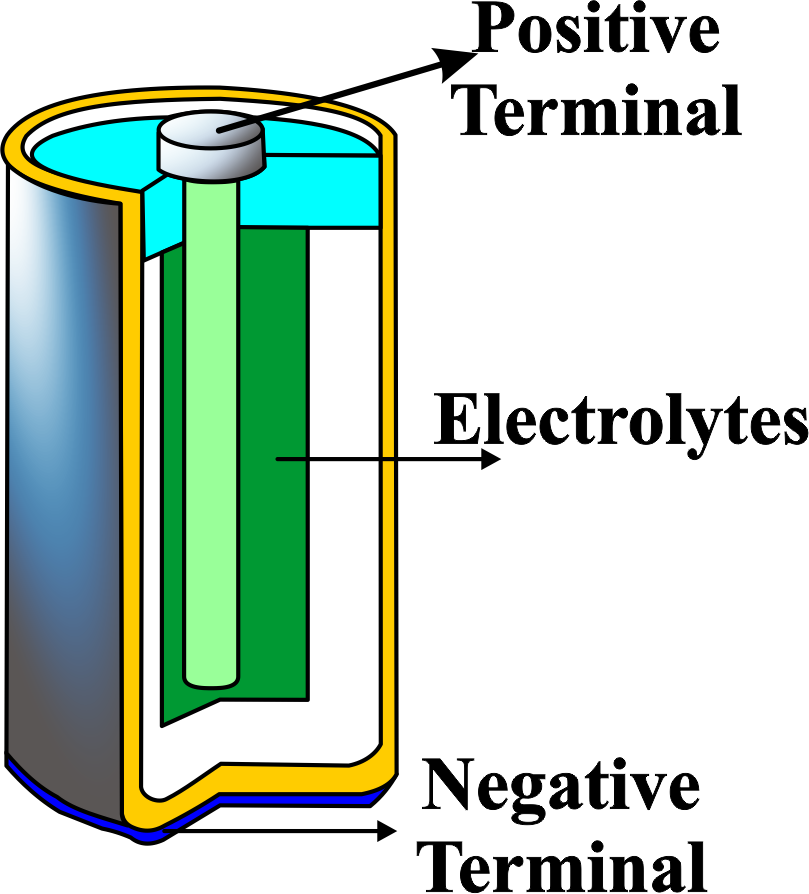
There are different types of electric cells, such as primary cells, secondary cells, fuel cells, and solar cells. Primary cells are disposable and cannot be recharged, such as dry cells and button cells. Secondary cells are rechargeable and can be used multiple times, such as lead-acid batteries and lithium-ion batteries. Fuel cells are cells that use hydrogen and oxygen as electrodes and produce water as a byproduct, such as in some vehicles and spacecraft.
Solar cells are cells that use light as an energy source and convert it into electricity, such as in solar panels and calculators.
concepts related to electric cell
Some of the basic concepts related to electric cell.
- Electric cell: A device that converts chemical energy into electrical energy by using electrodes and an electrolyte.
- Electrode: A conductor that participates in a chemical reaction with the electrolyte. An electric cell has two electrodes: a positive electrode called the cathode and a negative electrode called the anode.
- Electrolyte: A substance that can conduct electricity by producing ions. The electrolyte enables the flow of charge between the electrodes.
- Separator: A material that prevents direct contact between the electrodes and avoids short-circuiting.
- Electric current: The flow of electrons from the anode to the cathode through an external circuit. The electric current is driven by the potential difference between the electrodes.
- Chemical reaction: The process that occurs between the electrodes and the electrolyte, which produces new substances and releases or consumes electrons. The chemical reaction determines the type and capacity of the electric cell.
- Electromotive force (EMF): The maximum potential difference between the electrodes when the electric cell is not connected to any circuit. The EMF is a measure of the energy supplied by the electric cell per unit charge.
- Internal resistance: The resistance offered by the electric cell to the flow of electric current. The internal resistance depends on the materials and dimensions of the electrodes and the electrolyte. The internal resistance reduces the effective voltage and power output of the electric cell.
working of an electric cell
The working of an electric cell can be explained as follows:
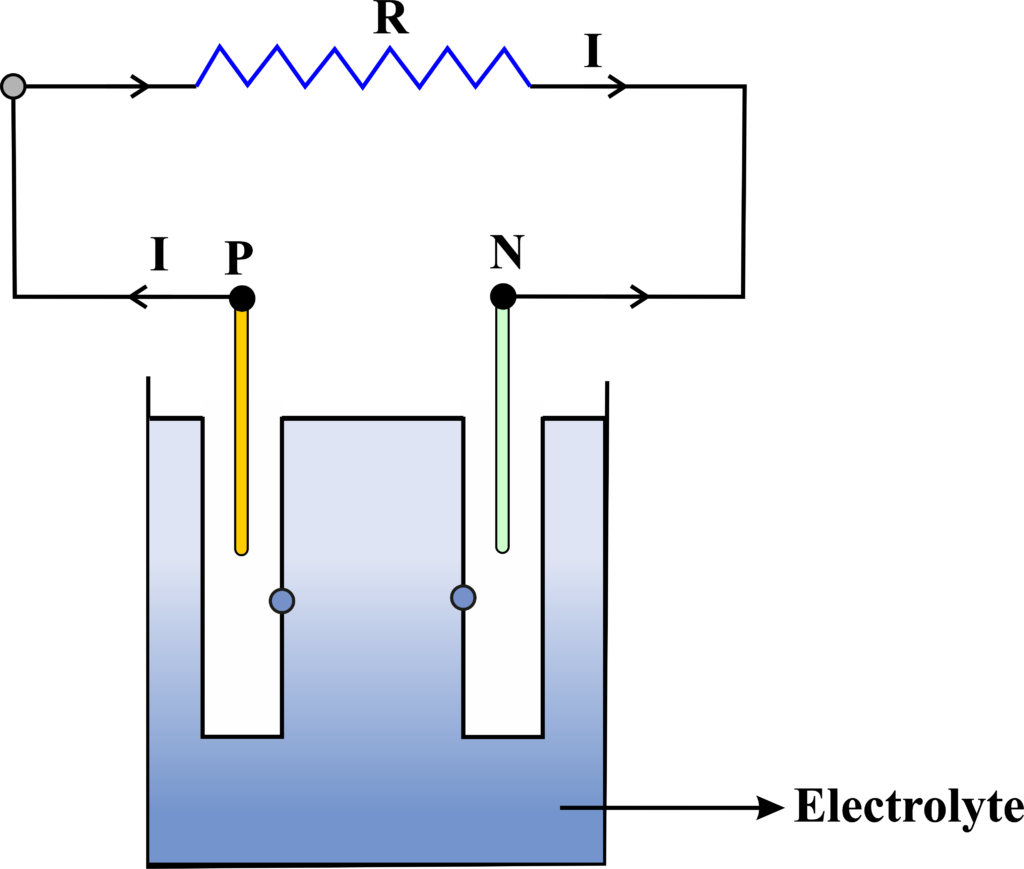
- An electric cell consists of two electrodes, a positive electrode called the cathode and a negative electrode called the anode, immersed in an electrolyte, which is a substance that can conduct electricity by producing ions.
- When the electrodes are connected to an external circuit, a chemical reaction takes place between the electrodes and the electrolyte. This reaction is called an oxidation-reduction reaction, or a redox reaction because it involves the transfer of electrons between the reactants.
- At the anode, the electrode material loses electrons and becomes oxidized. The electrons flow from the anode to the cathode through the external circuit. This is the electric current that powers the device connected to the cell.
- At the cathode, the electrode material gains electrons and becomes reduced. The electrons combine with the ions from the electrolyte and form new substances. The electrolyte also provides a path for the ions to move between the electrodes and complete the circuit.
- The chemical reaction between the electrodes and the electrolyte also produces a potential difference, or a voltage, between the electrodes. This voltage is the driving force for the electric current. The maximum voltage that a cell can produce is called the electromotive force, or the EMF, of the cell.
- The chemical reaction also causes the cell to lose its ability to produce electric current over time. This is because the reactants are consumed and the products accumulate, which reduces the potential difference and the electric current. This is why electric cells eventually run out of energy and need to be replaced or recharged.
Types of Electric Cell
Electric cells are devices that convert chemical energy into electrical energy or vice versa. Based on the rechargeable and non-rechargeable type, electric cells can be divided into two categories:
- Primary cells: These are electric cells that cannot be recharged once they are used up. They produce electric current from the spontaneous chemical reactions between the electrodes and the electrolyte. Examples of primary cells are dry cells, button cells, and zinc-carbon cells. Primary cells are usually cheap, simple, and disposable. They are used for low-power applications such as toys, clocks, and flashlights.
- Secondary cells: These are electric cells that can be recharged by reversing the chemical reactions that occur in them. They require an external source of electric current to restore the original state of the electrodes and the electrolyte. Examples of secondary cells are lead-acid batteries, nickel-cadmium batteries, and lithium-ion batteries. Secondary cells are usually more expensive, complex, and durable. They are used for high-power applications such as vehicles, laptops, and phones.
Primary cells
A primary cell is a type of battery that is designed to be used once and discarded, and not recharged with electricity and reused like a secondary cell. In general, the electrochemical reaction occurring in the cell is not reversible, rendering the cell unrechargeable. Examples of primary cells are dry cells, button cells, and zinc-carbon cells.
Some of the advantages of primary cells are:
- They are small in size, light in weight, and easy to use.
- They have longer service per charge and no risk of leakage of chemicals.
- They are cheap, easily available, and can be transported without much difficulty.
- They are used in portable electronic devices such as toys, clocks, and flashlights.
Some of the disadvantages of primary cells are:
- They cannot be recharged once they lose their electrical power and have to be thrown away.
- They are not ideally suited for heavy loads and high-power applications.
- They are highly toxic and produce a lot of waste when disposed of, which can harm the environment.
- They have a limited shelf life and may lose their capacity over time.
Primary cells are used for various purposes, such as:
- Medical devices: Primary cells are used to power devices such as pacemakers, hearing aids, and defibrillators, which require reliable and long-lasting power sources.
- Military applications: Primary cells are used to power devices such as radios, night vision goggles, and GPS systems, which require high performance and durability.
- Emergencies: Primary cells are used to power devices such as flashlights, radios, and smoke detectors, which are essential for safety and survival.
- Consumer electronics: Primary cells are used to power devices such as cameras, calculators, and remote controls, which are convenient and inexpensive.
Secondary cells
Secondary cells are electric cells that can be recharged by passing an electric current in the opposite direction of the discharge current. They have reversible chemical reactions between the electrodes and the electrolyte. Examples of secondary cells are lead-acid batteries, nickel-cadmium batteries, and lithium-ion batteries.
Some of the advantages of secondary cells are:
- They can be reused many times, which reduces the environmental impact and the cost of replacing them.
- They have better power output capabilities than primary cells and are suitable for high-power applications.
- They have lower internal resistance and higher efficiency than primary cells.
Some of the disadvantages of secondary cells are:
- They have higher initial costs than primary cells and require special chargers and maintenance.
- They have lower energy density and faster self-discharge than primary cells and may lose their capacity over time.
- They may suffer from memory effect, which reduces their performance if they are not fully discharged before recharging.
Some of the uses of secondary cells are:
- Car starters, electric vehicles, and hybrid vehicles, which require high power and durability.
- Portable devices such as laptops, phones, and cameras, which require long-lasting and reliable power sources.
- Backup power systems such as uninterruptible power supplies and battery storage power stations, which provide stability and security for the electrical grid.
The internal resistance of a cell
The internal resistance of a cell is the resistance offered by the cell itself to the flow of current. It is caused by the electrolytic material and the electrodes of the cell. The internal resistance of a cell reduces the effective potential difference and the current of the cell. The internal resistance of a cell is measured in ohms (Ω).
The internal resistance of a cell depends on several factors, such as the nature and concentration of the electrolyte, the temperature, the size and shape of the electrodes, and the age of the cell. The internal resistance of a cell can be calculated by using the formula:
\(\displaystyle r = \frac{E – V}{I}\)
where r is the internal resistance, E is the emf, V is the terminal voltage, and I is the current of the cell.
For example, if a cell has an emf of 1.5 V and a terminal voltage of 1.2 V when connected to a circuit with a current of 0.5 A, then the internal resistance of the cell is:
\(\displaystyle r = \frac{E – V}{I} = \frac{1.5 V – 1.2 V}{0.5 A} = 0.6 Ω\)
Potential Difference and EMF of a Cell
The potential difference of a cell is the voltage across its terminals when it is connected to a circuit. It is equal to the emf of the cell minus the voltage drop due to the internal resistance of the cell. The potential difference of a cell is measured in volts (V).
The emf of a cell is the maximum potential difference between its terminals when no current flows through the circuit. It is equal to the work done by the cell to move a unit charge from one terminal to the other. The emf of a cell is determined by the chemical reaction that occurs inside the cell. The emf of a cell is measured in volts (V).
The internal resistance of a cell reduces the effective potential difference and the current of the cell. The maximum current flow through a cell is the current that flows when the cell is short-circuited, i.e. when the external resistance of the circuit is zero. The maximum current flow through a cell is equal to the ratio of the emf of the cell and the internal resistance of the cell. The maximum current flow through a cell is measured in amperes (A).
The formulas for these quantities are:
\(\displaystyle V = E – Ir\)
\(\displaystyle E = \frac{W}{q}\)
\(\displaystyle I_{max} = \frac{E}{r}\)
where V is the potential difference, E is the emf, I is the current, r is the internal resistance, W is the work done, and q is the charge.
The cell is an important source of electrical energy in current electricity, which is the study of the flow of electric charges in a circuit. The cell provides the driving force for the current, and its emf determines the maximum potential difference that can be obtained in the circuit. The cell also has an internal resistance, which is the resistance offered by the cell itself to the current. The internal resistance reduces the effective potential difference across the cell, and it depends on the nature and concentration of the electrolyte, the temperature, and the age of the cell.
Electric cells have many applications in various fields, such as electronics, communication, transportation, medicine, and renewable energy. Electric cells power devices such as phones, laptops, cameras, and flashlights. Electric cells enable wireless communication such as radios, televisions, and satellites. Electric cells provide alternative sources of energy for vehicles such as electric cars, bikes, and trains. Electric cells support medical equipment such as pacemakers, hearing aids, and defibrillators. Electric cells also contribute to environmental sustainability by reducing greenhouse gas emissions and fossil fuel consumption.